X-ray imaging technology has been a cornerstone of scientific research and industrial applications for decades. From medical diagnostics to material analysis, X-ray imaging plays a crucial role in studying the internal structures of objects in a non-destructive manner. In recent years, technological advancements have significantly enhanced the resolution, sensitivity, and efficiency of laboratory X-ray imaging equipment, enabling groundbreaking discoveries in fields such as materials science, nanotechnology, and biomedical research.
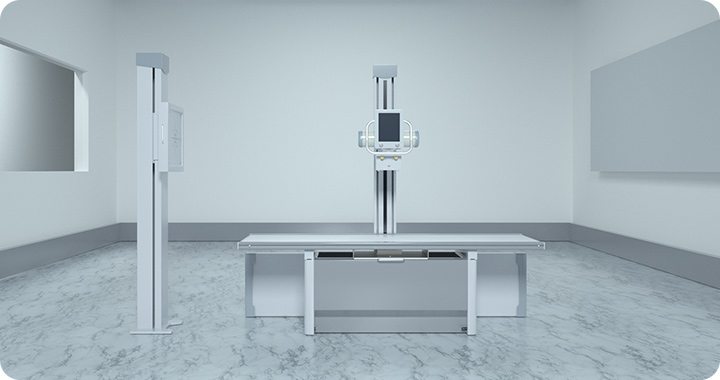
Key Technological Innovations in X-ray Imaging Equipment
High-Resolution X-ray Imaging
One of the most significant advancements in laboratory X-ray imaging is the improvement in resolution. Traditional X-ray imaging systems often struggled with limited resolution due to detector constraints and X-ray source limitations. Recent developments have led to:
Micro- and Nano-CT (Computed Tomography): These systems use high-brilliance X-ray sources and advanced optics to achieve resolutions down to the nanometer scale, enabling detailed imaging of microstructures in biological and material samples.
Phase-Contrast and Coherent X-ray Imaging: Traditional X-ray imaging relies on absorption contrast, but phase-contrast techniques enhance image quality by detecting subtle variations in phase shifts, allowing for the visualization of soft tissues and low-density materials with unprecedented clarity.
Advanced X-ray Sources
The development of new X-ray sources has significantly improved imaging capabilities.
Synchrotron Radiation X-ray Sources: These high-intensity X-ray sources, available in advanced research facilities, provide ultra-bright, tunable X-ray beams for high-resolution imaging and spectroscopy.
Compact X-ray Sources: Laboratory-scale high-brilliance X-ray sources, such as laser-driven or plasma-based sources, offer improved performance while reducing the reliance on large synchrotron facilities.
Monochromatic and Tunable X-ray Sources: Advanced X-ray tubes with monochromators allow researchers to select specific X-ray wavelengths, enhancing contrast and reducing radiation dose.
Enhanced X-ray Detectors
Modern X-ray detectors have seen significant improvements in sensitivity, speed, and resolution.
Photon-Counting Detectors: Unlike traditional charge-coupled device (CCD) or flat-panel detectors, photon-counting detectors measure individual X-ray photons, providing superior signal-to-noise ratio, energy discrimination, and reduced image artifacts.
High-Speed Detectors: Innovations in CMOS-based X-ray detectors have enabled real-time imaging at high frame rates, which is essential for capturing dynamic processes such as in-situ material deformation or biological tissue dynamics.
Multi-Energy and Spectral Imaging: Advanced detectors can capture multiple energy levels simultaneously, allowing for enhanced material differentiation and improved contrast in imaging.
Artificial Intelligence and Computational Imaging
AI-driven techniques are revolutionizing X-ray imaging by enhancing image reconstruction, analysis, and interpretation.
Deep Learning-Based Image Reconstruction: AI algorithms improve image quality by reducing noise, enhancing contrast, and enabling super-resolution imaging.
Automated Defect Detection: In industrial and biomedical applications, AI models can automatically detect defects, tumors, or structural anomalies, reducing the need for manual analysis.
3D and 4D Image Processing: Machine learning algorithms facilitate high-speed 3D image reconstruction and time-resolved (4D) imaging, which are critical for studying dynamic processes in materials and biological systems.
Non-Destructive and In-Situ Imaging Techniques
Modern X-ray imaging systems are increasingly used for real-time, non-destructive testing in research and industry.
In-Situ Imaging of Material Changes: Advances in X-ray microscopy allow researchers to observe structural changes in materials under various conditions such as heat, pressure, or chemical reactions.
Time-Resolved X-ray Imaging: High-speed imaging techniques enable researchers to capture transient events, such as crack propagation in materials or cellular responses in biological tissues.
X-ray Diffraction and Spectroscopy Integration: Combining X-ray imaging with diffraction and spectroscopy techniques provides comprehensive insights into material composition and structural properties.
Recent Advances and Applications in Different Fields
Biomedical Research
High-Resolution Imaging of Soft Tissues: Phase-contrast X-ray imaging has significantly improved the visualization of soft tissues, aiding in cancer research and regenerative medicine studies.
Micro-CT for Cellular and Tissue Analysis: Advances in micro-CT imaging allow for detailed structural analysis of biological tissues and even single cells.
AI-Driven Medical Diagnostics: AI-assisted X-ray imaging helps in early disease detection, improving diagnostic accuracy in conditions such as osteoporosis and lung diseases.
Materials Science and Engineering
Nano-CT for Microstructural Analysis: High-resolution X-ray tomography enables scientists to study the internal microstructures of advanced materials such as composites, metals, and ceramics.
In-Situ Mechanical Testing: X-ray imaging systems are increasingly used to observe real-time material deformation and fracture mechanisms.
Battery and Energy Storage Research: X-ray imaging plays a crucial role in analyzing the internal structures of lithium-ion batteries, fuel cells, and other energy storage devices.
Industrial and Aerospace Applications
Non-Destructive Testing (NDT): High-resolution X-ray imaging ensures the integrity of aerospace components, electronic circuits, and manufacturing parts without damaging them.
Additive Manufacturing (3D Printing) Quality Control: X-ray imaging helps detect defects in 3D-printed parts, ensuring product reliability and consistency.
Cultural Heritage and Archeology: X-ray imaging is used for non-invasive analysis of ancient artifacts, paintings, and fossils, revealing hidden details without harming delicate specimens.
Future Trends and Challenges
Miniaturization and Portability
Future X-ray imaging systems are expected to become more compact and portable, allowing for greater accessibility in field research, remote medical diagnostics, and industrial inspections.
Integration with Multi-Modal Imaging
Combining X-ray imaging with other imaging techniques such as MRI, PET, and optical microscopy will provide more comprehensive data for complex biological and material studies.
Reducing Radiation Exposure
Advances in detector sensitivity and AI-driven noise reduction will allow for high-quality imaging with lower radiation doses, making X-ray imaging safer for patients and researchers.
Higher Resolution and Faster Imaging
The push for higher resolution and real-time imaging capabilities will continue, with the development of next-generation X-ray sources, improved detector technology, and advanced computational imaging methods.
The rapid advancements in laboratory X-ray imaging equipment are transforming scientific research and industrial applications. From high-resolution imaging and AI-driven analysis to real-time in-situ testing and multi-modal integration, these innovations are expanding the capabilities of X-ray imaging beyond traditional boundaries. As technology continues to evolve, X-ray imaging will remain an essential tool for understanding the internal structures of materials, biological tissues, and industrial components, paving the way for new discoveries and improved applications across multiple disciplines.